Klaus Lackner: Negative emissions: Cleaning up climate change
Prof. Klaus S. Lackner, Director of Center for Negative Carbon Emissions and Professor at the School of Sustainable Engineering and the Built Environment of the Ira A. Fulton Schools of Engineering, Arizona State University, AZ, USA. Director of the Lenfest Center for Sustainable Energy, The Earth Institute, Columbia University, NY, USA.
If we want to clean-up emissions as well as progress global development, Klaus Lackner has solutions. He steps this out, reflects on changing the sequestration debate and helps to explain what it is going to take to balance the global carbon budget.
Carbon dioxide emitted in the energy sector is the main driver of climate change.[i] This needs to stop.[ii] Energy efficiency and energy conservation will help, but modern societies rely on energy.[iii] Expensive energy would stymie progress in the developing world. Delayed or frustrated development would prevent countries from reaching the standard of living necessary for halting rapid population growth, which presents an even more serious challenge to sustainability than climate change. Lack of energy will make it more difficult to provide food, water and mineral resources, and hinder environmental cleanup. Therefore, the discussion cannot be about stopping energy consumption but must be about cleaning up the energy sector.
Today’s energy infrastructure, which is based on fossil fuels and simply dumps the CO2 byproduct into the atmosphere, is not sustainable. New energy systems must be carbon-neutral. This means moving away from fossil carbon while embracing a waste management paradigm for the remaining emissions.[iv] Few energy resources could work sustainably on a global scale, currently 18 TW of primary energy. Only solar energy, nuclear energy and possibly fossil carbon with carbon capture and storage can operate at such scales. With photovoltaic energy costs dropping rapidly [REF], a transition to a carbon-neutral world may already be underway, but the CO2 emitted before a complete transition cannot be ignored.[v] The world will need to clean up the mess it already made. Based on current energy use, future fossil emissions will need to cleaned up for decades to come.
Once mobilized, carbon stays in atmosphere/ocean/biosphere system for tens of millennia. For the first hundred years half of the carbon remains in the atmosphere. The rest acidifies the ocean and eutrophicates the biosphere [REF]. Mitigation is good but insufficient. “Reduce, reuse, recycle” is a great call to arms, but does not give permission to dump the remaining CO2. Zero waste is a noble goal, but until it is attained CO2 must be disposed of. This will require big political change, but it may prove easier than forcing lifestyle changes[vi],[vii] In the end, for every ton of carbon coming out of the ground another ton will have to be disposed of. This way the world’s carbon budget can be balanced.
How much CO2 will have to be recovered? Climate targets are given as temperature increase. For a long time, the limit stood at 2°C. After Paris in 2015, the new goal is 1.5°C. Modelers suggest that warming is linear in cumulative CO2 emissions, and that 1 ppm causes about 0.01°C heating. The 2°C limit would be breached around 480 ppm; 1.5°C around 430 ppm. This translation is subject to uncertainties. For example, the last assessment report states on page 20:[viii] “Emissions scenarios leading to CO2-equivalent concentrations in 2100 of about 450 ppm or lower are likely to maintain warming below 2°C over the 21st century relative to pre-industrial levels.” An associated footnote states that the total greenhouse gas concentration in 2011 reached 430 ppme. Given the CO2 additions since 2011 this line is being crossed now. In other words, the likelihood of exceeding 2°C ceases to be low. On the other hand, the recent report on 1.5 degree states: “Past emissions alone are unlikely to raise global-mean temperature to 1.5°C above pre-industrial levels …” (Page 51).2
The modeler’s consensus suggests that temperatures will stop rising if emissions stop. This requires a subtle cancellation of ocean warming and CO2 transfer into the ocean [REF]. Others, like Jim Hansen, have stated that CO2 levels should be kept below 350 ppm [REF], in which case we already face a large overdraft of the carbon budget. At current rate of emission, we will hit 450 ppm in 16 years. Therefore, the necessary carbon reductions could easily exceed 100 ppm. Figure 1 (below), using a very simple model, shows how difficult it will be to avoid an overshoot, even if there is some carbon budget left.
At this point, it is nearly impossible to avoid a serious overshoot in CO2. While the best solution to the climate change problem is to phase out fossil fuels, it will come too late for the 2°C limit. The process should be accelerated, but sudden transitions also pose risks. Since the world has entered an overshoot scenario, we have to return as fast as possible to safe levels. This requires negative emissions. Once negative emissions are introduced, one can set even lower targets. Rather than aiming for 450 ppm or 500 ppm, one can ask whether 350 ppm or the 280 ppm would be better choices. Regardless of the target, the amount of CO2 to be recovered is likely very large. The IPCC estimates numbers around 10 to 15 Gt CO2 annually [REFs]. Even these numbers sound low. Recovering 100 ppm over 40 years would require an average negative emission rate of 2.5 ppm or about 35 to 40 Gt per year.
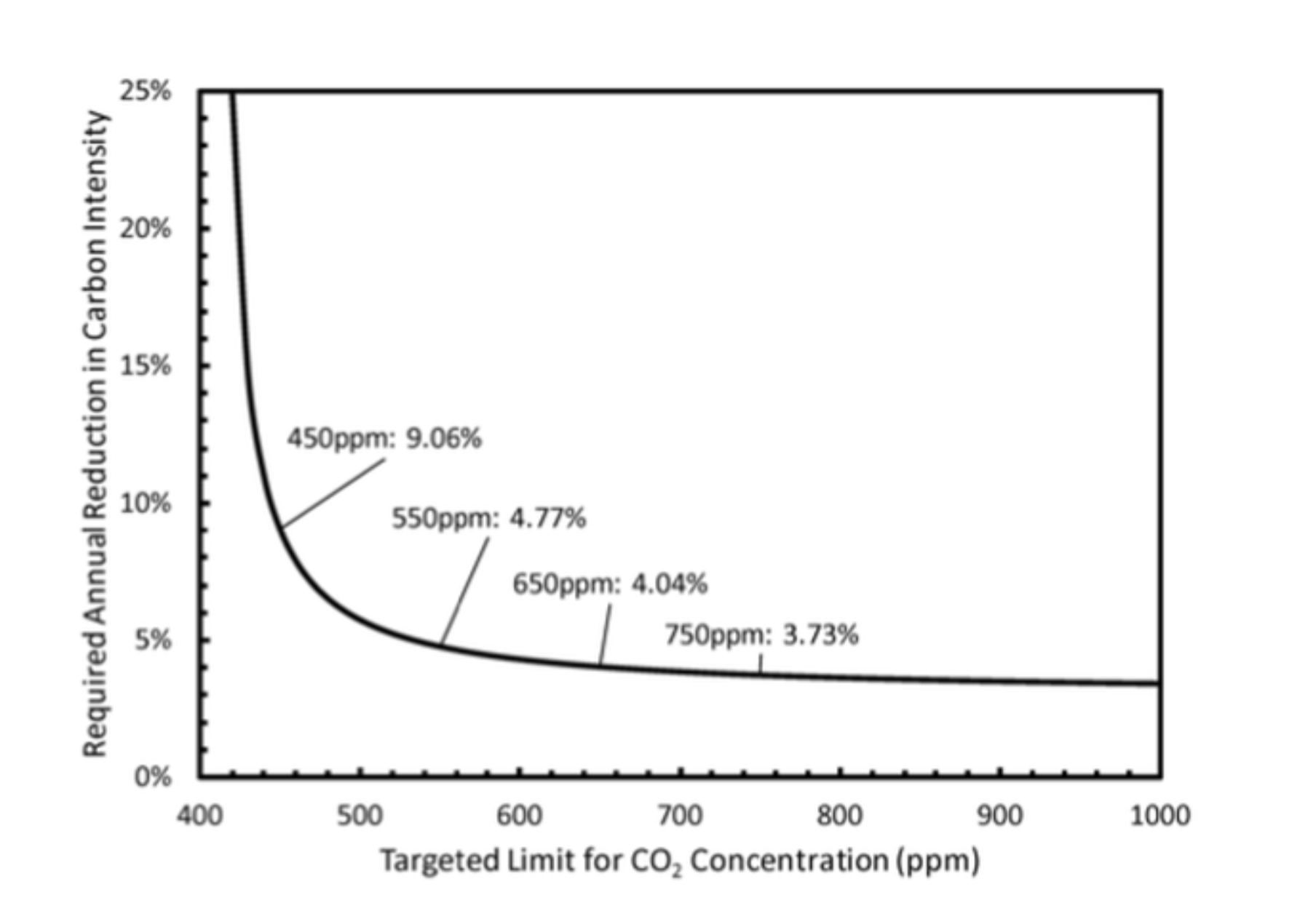
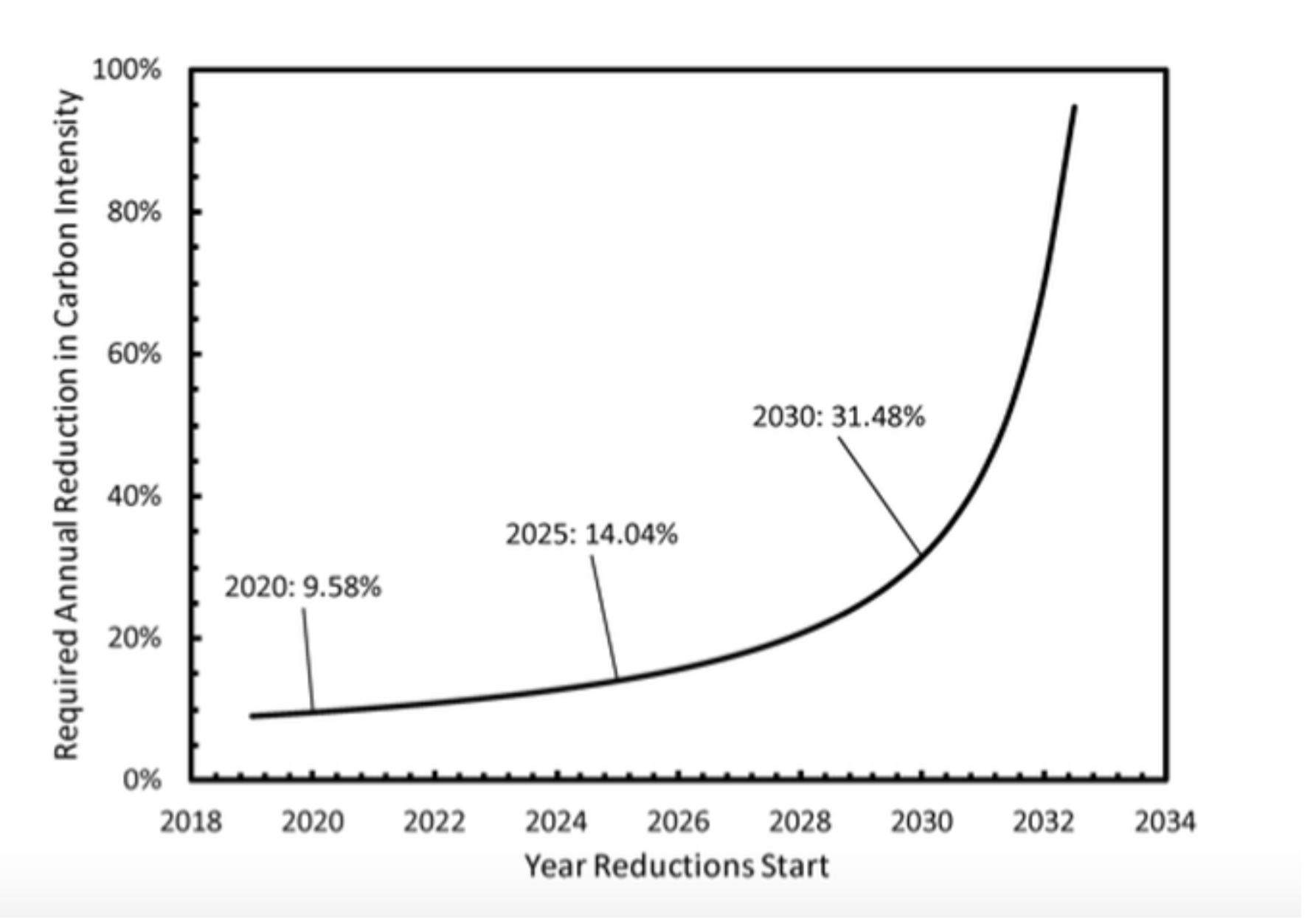
Figure 1:
The following simple model illustrates the challenge of stopping climate change in time. We measure emissions by the rate of increase of CO2 in the atmosphere and assume an annual rise in these emissions until action is taken at some future time. After that, emissions will shrink every year by a fixed percentage. As a result, CO2 concentrations in the air will rise asymptotically to final value. Starting 2019 with 410 ppm in the air, a rate of increase of 2.5ppm/yr, a world growth of emissions of 2% per annum, and a world GDP growth of 3% per annum, we can calculate peak CO2 based on the time action starts, and the annual reduction in carbon intensity. (Carbon intensity is the ratio of CO2 emissions to GDP; the reduction in carbon intensity exceeds CO2 emission reductions by the economic growth rate assumed to be 3%.) Panel (a) shows the annual reduction required if action starts in 2019 as function of the desired final value. Panel (b) assumes that the desired limit is 450 ppm, but that action is delayed to the year indicated on the abscissa. Historically, efficiency improvements have lowered the carbon intensity by about 1% per annum. Reductions of 10% or more, are nearly impossible to achieve, but are in line with the conclusions of the IPCC report on 1.5C warming.
Options for Negative Emissions
Negative emissions require technologies for removal from or fixation of carbon in the environment, i.e., the biosphere, surface ocean, or the atmosphere. Since these three carbon reservoirs are tightly coupled, removing or fixating carbon in one reservoir will also lower the excess carbon in the others.
Growing forests, increasing stable biocarbon in the soil, or exporting biomass from the surface ocean to depth reduce excess mobile carbon in the biosphere. Growing and harvesting biomass for conversion into CO2 or other forms of carbon that can be safely stored taps storage reservoirs which are far larger than the biomass itself. Living biomass holds about 60 years of current emissions. Unfortunately, biomass production requires large areas, enough to provide the sun-shine energy for converting CO2 into biomass. With a conversion efficiency of intensive agriculture ~1%, the area requirement for pulling back 100 ppm over forty years would exceed the world’s agricultural land. It would collide head-on with food production and pose severe environmental challenges.
Collection from the ocean by technical means is thermodynamically equivalent to removing CO2 from air, because the two reservoirs are in equilibrium. Ocean water holds about 2 mol/m3 of CO2 rather than the 0.016 mol/m3 in air. However, in air 1 : 2500 molecules is a CO2. In Ocean water it is 1 : 25000. For most processes this ratio is more relevant than the density, and therefore we placed our effort on air capture. Another advantage of air capture is that the air mixes so fast that all locations on the planet are equivalent for collection. In theory, one could concentrate all collectors in one remote site. Both, ocean capture and air capture are scalable and without investment limitations could remove all excess CO2 in a decade or two.
Carbon management requires carbon storage for negative emissions, fuel and chemical synthesis for a closed carbon cycle, and requires carbon removal with a focus on direct air capture. A substantial fraction of the carbon released from energy consumption will end up in the environment, even if many point sources can be mitigated by other means. Carbon storage technologies have been developed over the last few decades, and there seems to be a general consensus that combinations of geological storage and mineral sequestration have the capacity to store all the excess carbon humans can produce. Geological storage in particular is affordable. Scaling has not yet been demonstrated, simply because there was no need for it in the past.
Fuel synthesis including electrolysis, water gas shift, Fischer Tropsch, Sabatier and methanol production, were invented in the first half of the last century. Because of the low cost of fossil fuels, they never penetrated the market, except in rare circumstances where political constraints made workarounds necessary. For example, South Africa because it was embargoed introduced Fischer-Tropsch technology to convert syngas derived from coal into liquid fuels. The technology is still in use. Direct air capture has not been tested seriously, unless one counts the CO2 removal at air liquefaction plants. Of the critical technologies it is the least established and therefore in most need of development.
Air Capture is an enabling technology. It can take care of all emissions that were otherwise considered too difficult. Air capture combined with geological storage always provides an option and it therefore defines the marginal cost of carbon, even if many emissions can be avoided by more cost-effective means, including efficiency improvements and point source capture. Direct air capture can remove excess carbon from the environment. It can operate at the necessary scale to pay back the world’s carbon budget overdraft. It will, however, require vast storage capacity. Closing the carbon cycle in the transportation sector will require some form of air capture. The CO2 removed from the atmosphere can be converted back into synthetic fuel or disposed of permanently for a fossil carbon-based fuel. Fuel synthesis could take advantage of excess renewable energy, and thereby solve the intermittency problem associated with solar or wind energy.
In spite of its conceptual simplicity and strategic importance, it has been difficult to gain acceptance for direct air capture. It is caught between different established groups. For climate change deniers there is no need for it, and even many who are convinced of climate change have not yet accepted that CO2 once emitted persists for centuries. How else could one advocate for 350 ppm as 350.org has without accepting the need for negative emissions.
One of the most damaging arguments against negative emissions and direct air capture has been the “moral hazard” argument, which essentially sees the ability to create a negative emission as a threat to mitigation and a phase out of fossil fuels. I would argue to the contrary direct air capture offers a way out and therefore is likely to accelerate action. Moreover, at this point any delay in rolling out negative emissions will increase the by now unavoidable damages.
The owners of oil and oil production infrastructure also have shown no interest, even though their societal license to operate will be lost once the dangers of climate change are fully understood. The fear that one would be called to action if air capture technology is available, should be more than balanced by the concern that customers will be abandoning transportation fuels if they cannot be rendered carbon-neutral. Oil companies through their inaction may already have ceded the automobile sector, which represents about 40% of world oil consumption. Sarewitz and Nelson noted that air capture makes a good technological fix, because it embodies the cause and effect relationship for the solution, can be easily quantified and provides a standardized technology that can be improved by research.
Feasibility of Air Capture
Air capture is technically feasible. The remaining concern is that the technology will remain too expensive, because the CO2 concentration in the air is too low. The concern is summarized by Sherwood’s Rule, which states that separation costs of related technologies scale linearly with dilution (Fig. 2). If Sherwood’s Rule is applicable, CO2 capture from air should be 300 times as expensive from flue gas, and therefore would be uneconomic. However, Sherwood’s Rule is not ironclad. It is rooted in the observation that for most separation processes the first step, which needs to touch the entire input stream dominates costs. This applies to metals which cost about $10 per ton of ore. This budget only leaves room for mining, crushing, and grinding the ore, running a flotation and disposing of the tailings. Apparently, downstream processing costs of the concentrate are negligible by comparison. This explains Sherwood’s Rule.
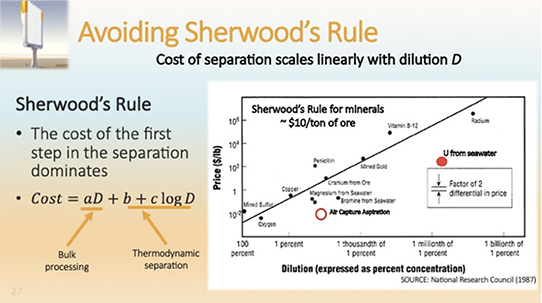
Figure 2: Sherwood’s Rule as Exemplified in the old Reports
However, there are exceptions. For example, Sherwood’s Rule breaks down for the extraction of uranium from seawater, which at 3 parts per billion is far more daunting than the extraction of CO2 from air. Certain resins selectively bind uranium and can be processed for their uranium content. Initial attempts failed because the cost of pumping water through filters was too expensive. It is this term which represents Sherwood’s linear contribution. Japanese researchers avoided pumping costs by introducing artificial kelp, a form of the resin which is passively exposed to ocean currents while loading up with uranium. By eliminating nearly all contacting cost, this approach successfully broke Sherwood’s Rule. The same is true for direct air capture. By standing passively in the wind like an artificial tree, we avoid nearly all costs that scale linearly with dilution. We are left with regeneration costs that scale logarithmically.
In short, carbon dioxide is not too dilute. Windmills mine air for kinetic energy. If we value kinetic energy at 5¢/kwh, a cubic kilometer of air moving at 6m/s contains $300 of value. If CO2 warrants a tipping fee of $30/t, the same cubic kilometer of air holds $21,000 of CO2. In short, CO2 is a much richer target than wind energy. Thermodynamic constraints also do not pose a serious obstacle. Regeneration energies are roughly twice as large as for flue gas scrubbers, but compared to the energy obtained from producing CO2, both are very small. While the combustion of gasoline releases 700 kJ/mol of CO2, the thermodynamic requirement of producing CO2 from air at 22 kJ is about twice as large as it is for extracting it from flue gas. Regeneration of the sorbent is therefore not subject to Sherwood’s Rule.
Today Air capture is real. A number of start up companies are trying to establish the technology. Air capture has clearly beaten Sherwood’s Rule and it has broken the $600/t barrier posited by the 2011 APS report [REF]. It still is too expensive, but the assumption that mass production can lower costs dramatically is highly plausible. The same argument has been made for wind and solar energy from the start. Cost reductions by two orders of magnitude have proven the assumption correct.
ASU's Air Capture Approach
Our own design for air capture relies on three principles. (1) It is passive; (2) it relies on a moisture swing sorbent; and (3) it aims to use mass production to drive down the cost of production and operation of air capture units. We are using an anionic exchange resin that has a high affinity for CO2 when it is dry and loses it when wet [REFS]. We raise the partial pressure of CO2 hundred-fold without spending significant energy. Instead we consume water which has a much lower cost. This moisture swing gets us to about 4 kPa of CO2, or energetically about half way to concentrated, pressurized CO2. Like everyone else, we spend energy in producing compressed CO2 but on a thermodynamic scale our starting point for spending energy is the half-way point for everyone else. There are multiple ways to accomplish this task, and they are all cheaper than extracting CO2 from flue gas, which has similar concentrations of CO2 than our first stage product.
We have run passive prototypes outside (Figure 3). We are now developing an advanced design for passive air capture that uses columns of stacked flat disks exposed to wind (Figure 4). If they operate with the moisture swing sorbent the loaded disks are dropped into a container where they are exposed to moisture to release the CO2.
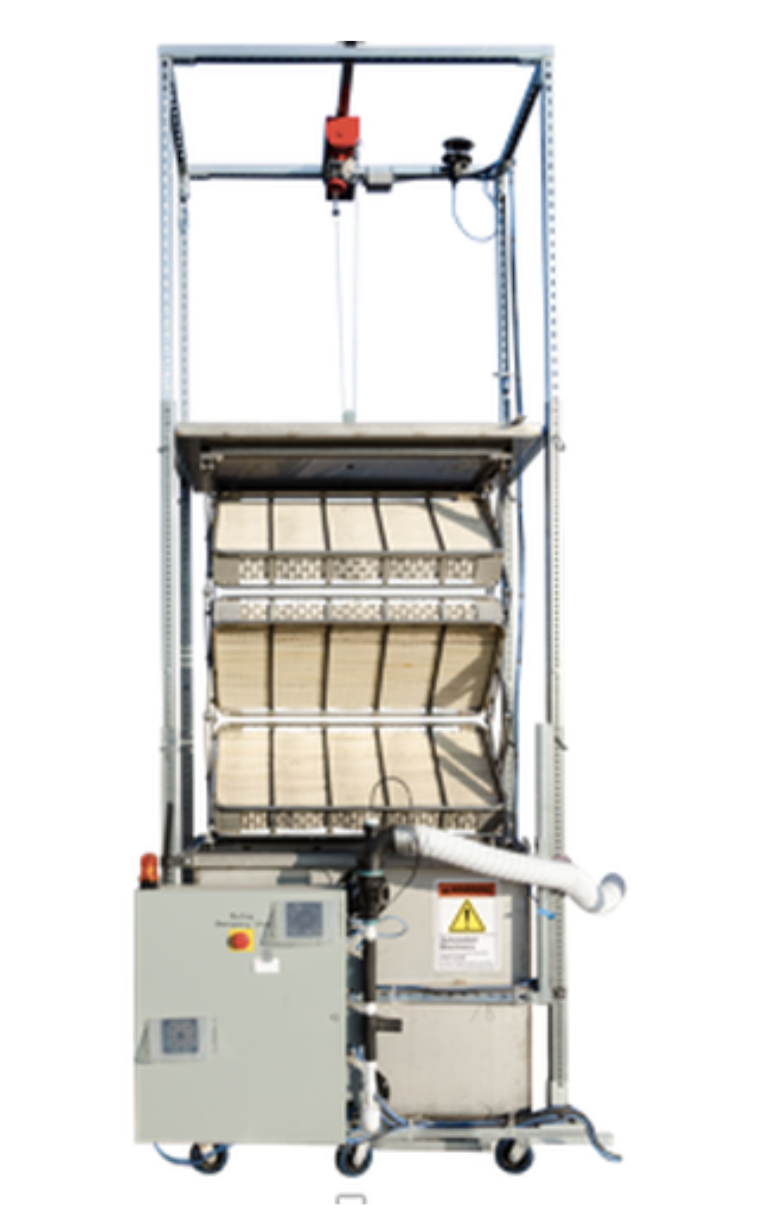
Figure 3: The ASU Prototype. Operated at Polytech Campus
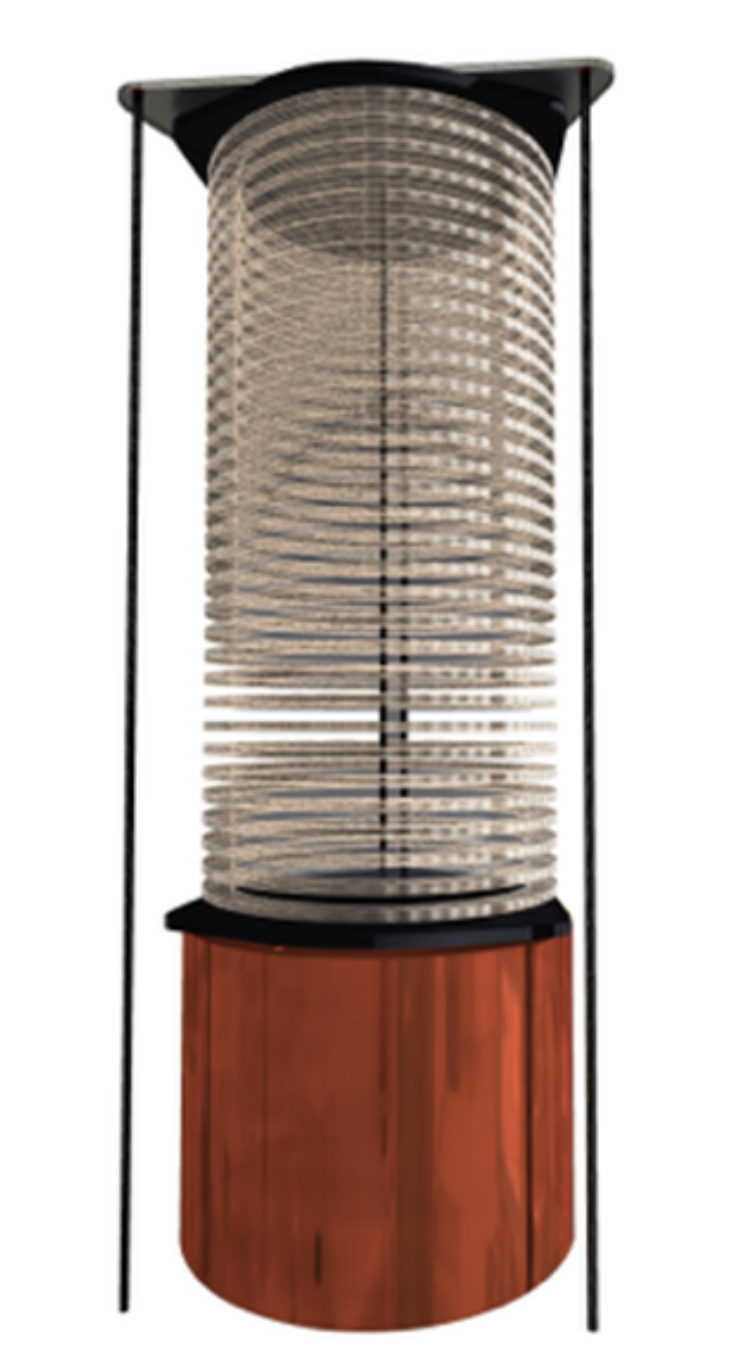
Figure 4: The Tiburio Design
The path to cost reduction is through mass production. In many industries the cost of production drops roughly 20% for every doubling in cumulative output [REFS]. If production increases 1000-fold, unit costs would drop 10-fold. Starting with a handful of units and aiming for 100s of millions, there is every reason to believe that cost will come down from the current few hundred dollars per ton to far below $100/ton.
If this can be accomplished direct air capture can make a big difference in carbon management. If air capture were tasked to remove 100 ppm in 40 years, it would have to operate 100 million 1-ton-per day units collecting 36 Gt/yr, each small enough to fit into a shipping container. To maintain this fleet with a ten-year turnover would require a production capacity of 10 million units per year. Production of cars and trucks amounts to 80 million units per year and Shanghai Harbor alone processes more shipping containers than could be filled by one year of production. There also is plenty of room to install that many units. On land there would need to be one every 3 km2, although in practice one would cluster them into large windfarms. Thus, scaling does not appear to pose serious challenges beyond the will and the resources to start a new large industry, which as a waste management industry could reach revenues of a trillion dollar per year.
Conclusions
Air capture is one of the few technologies that could reach full scale, combined with geological storage, it could put away 100 ppm of excess CO2 or even more if that proved to be necessary. Can it be done in time? On this question I am far less sanguine. Analogues with other technologies suggest that after invention there typically is a latency time, which is followed by rapid growth that results in ubiquitous deployment, maybe a decade or two later. If we optimistically assume that after a start 20 years ago [REF], the latency time is over and that growth starts now, we would take maybe 20 years to reach scale. Then it still takes 40 years to draw down the carbon, so one could reach the end of the overshoot near the end of the century, which leaves plenty of room for damages.
The really difficult question is how to get started. I believe that it will require voluntary action to set an example for politicians to follow. For example, oil companies could offer carbon neutral gasoline to consumer. The US government has expressed its interest in substituting the sequestration of air captured CO2 with a $50/t tax credit under a tax rule known as 45Q. Environmentally minded consumers, logistics companies or airlines could purchase carbon neutral fuel contributing $25/t of CO2. Oil companies should have a strategic interest to demonstrate that air capture is possible, because without it they will lose their societal license to operate. If costs of air capture and storage can be held below $100/t, their cost would be another $25/t. The premium on carbon-free gasoline for the volunteer would be 22¢/gallon or less than 10% of the price of gasoline. If 1% of customers volunteered, it would completely change the discussion on sequestration.
To learn more visit: Center for Negative Carbon Emissions (CNCE)
References
[i] Blanco G., R. Gerlagh, S. Suh, J. Barrett, H. C. de Coninck, C. F. Diaz Morejon, R. Mathur, N. Nakicenovic, A. Ofosu Ahenkora, J. Pan, H. Pathak, J. Rice, R. Richels, S. J. Smith, D. I. Stern, F. L. Toth, and P. Zhou, 2014: Drivers, Trends and Mitigation. In: Climate Change 2014: Mitigation of Climate Change. Contribution of Working Group III to the Fifth Assessment Report of the Intergovernmental Panel on Climate Change [Edenhofer, O., R. Pichs-Madruga, Y. Sokona, E. Farahani, S. Kadner, K. Seyboth, A. Adler, I. Baum, S. Brunner, P. Eickemeier, B. Kriemann, J. Savolainen, S. Schlömer, C. von Stechow, T. Zwickel and J.C. Minx (eds.)]. Cambridge University Press, Cambridge, United Kingdom and New York, NY, USA.
[ii] IPCC, 2018: Global Warming of 1.5°C. An IPCC Special Report on the impacts of global warming of 1.5°C above pre-industrial levels and related global greenhouse gas emission pathways, in the context of strengthening the global response to the threat of climate change, sustainable development, and efforts to eradicate poverty [V. Masson-Delmotte, P. Zhai, H. O. Pörtner, D. Roberts, J. Skea, P.R. Shukla, A. Pirani, W. Moufouma-Okia, C. Péan, R. Pidcock, S. Connors, J. B. R. Matthews, Y. Chen, X. Zhou, M. I. Gomis, E. Lonnoy, T. Maycock, M. Tignor, T. Waterfield (eds.)]. In Press.
[iii] Lackner, Klaus S. "Comparative impacts of fossil fuels and alternative energy sources." In Carbon Capture, pp. 1-40. 2009. Issues in Environmental Science and Technology, 29. Carbon Capture: Sequestration and Storage. Edited by R.E. Hester and R.M. Harrison. Royal Society of Chemistry 2010
[iv] Lackner, Klaus S., and Christophe Jospe. "Climate Change is a Waste Management Problem." Issues in Science and Technology 33, no. 3 (2017): 83-88.
[v] Lackner, Klaus S., Sarah Brennan, Jürg M. Matter, A-H. Alissa Park, Allen Wright, and Bob Van Der Zwaan. "The urgency of the development of CO2 capture from ambient air." Proceedings of the National Academy of Sciences 109, no. 33 (2012): 13156-13162.
[vi] Sarewitz, D. and Nelson, R., 2008. Three rules for technological fixes. Nature, 456(7224), p.871.
[vii] Lackner, Klaus S. "The promise of negative emissions." Science 354, no. 6313 (2016): 714-714.
[viii] IPCC, 2014: Climate Change 2014: Synthesis Report. Contribution of Working Groups I, II and III to the Fifth Assessment Report of the Intergovernmental Panel on Climate Change [Core Writing Team, R.K. Pachauri and L.A. Meyer (eds.)]. IPCC, Geneva, Switzerland, 151 pp.